Engineers create device that generates electricity out of thin air and needs no direct sunlight
The device, called a thermal resonator, could likely provide long-lasting, low-power energy sources for space rovers exploring other planets.
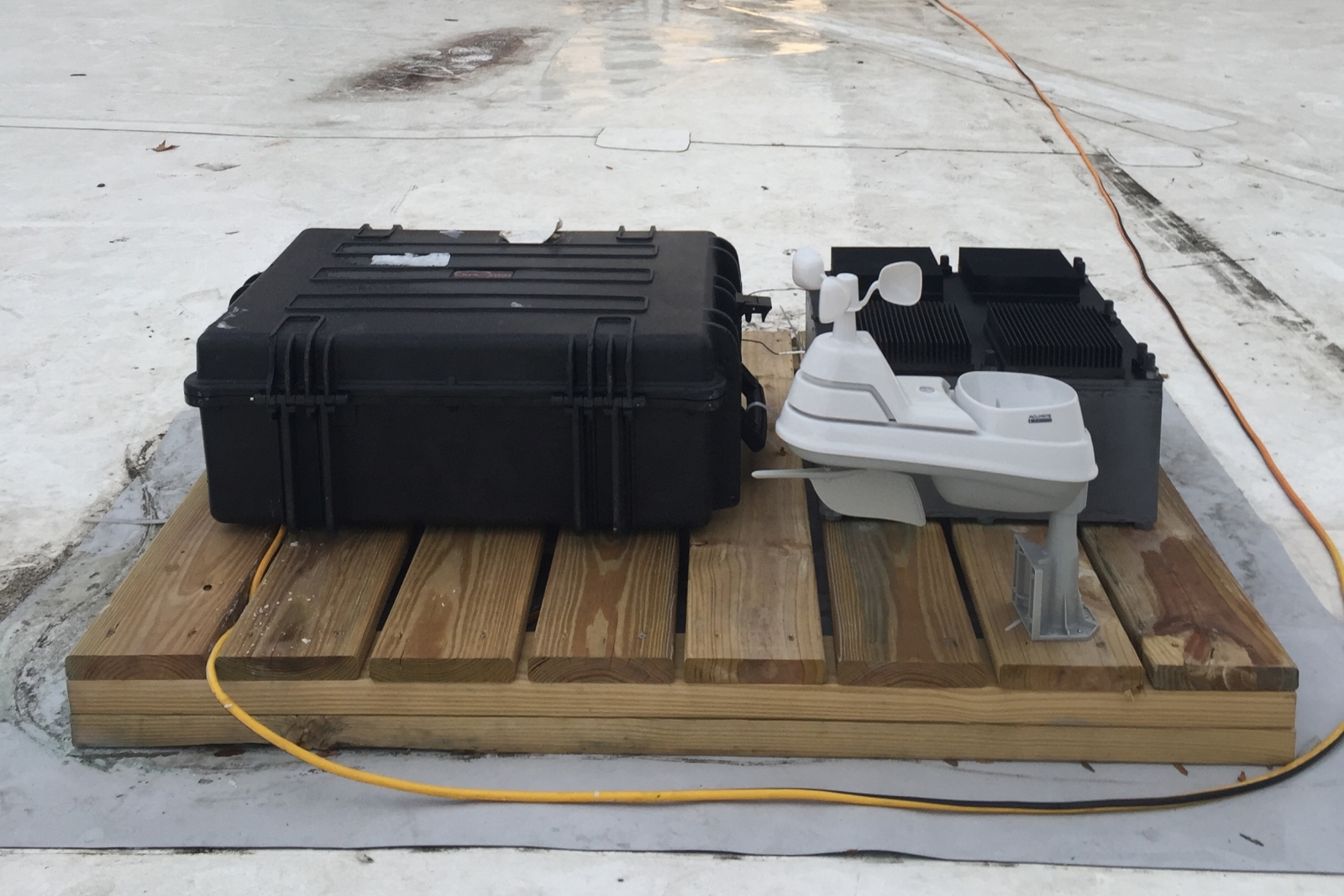
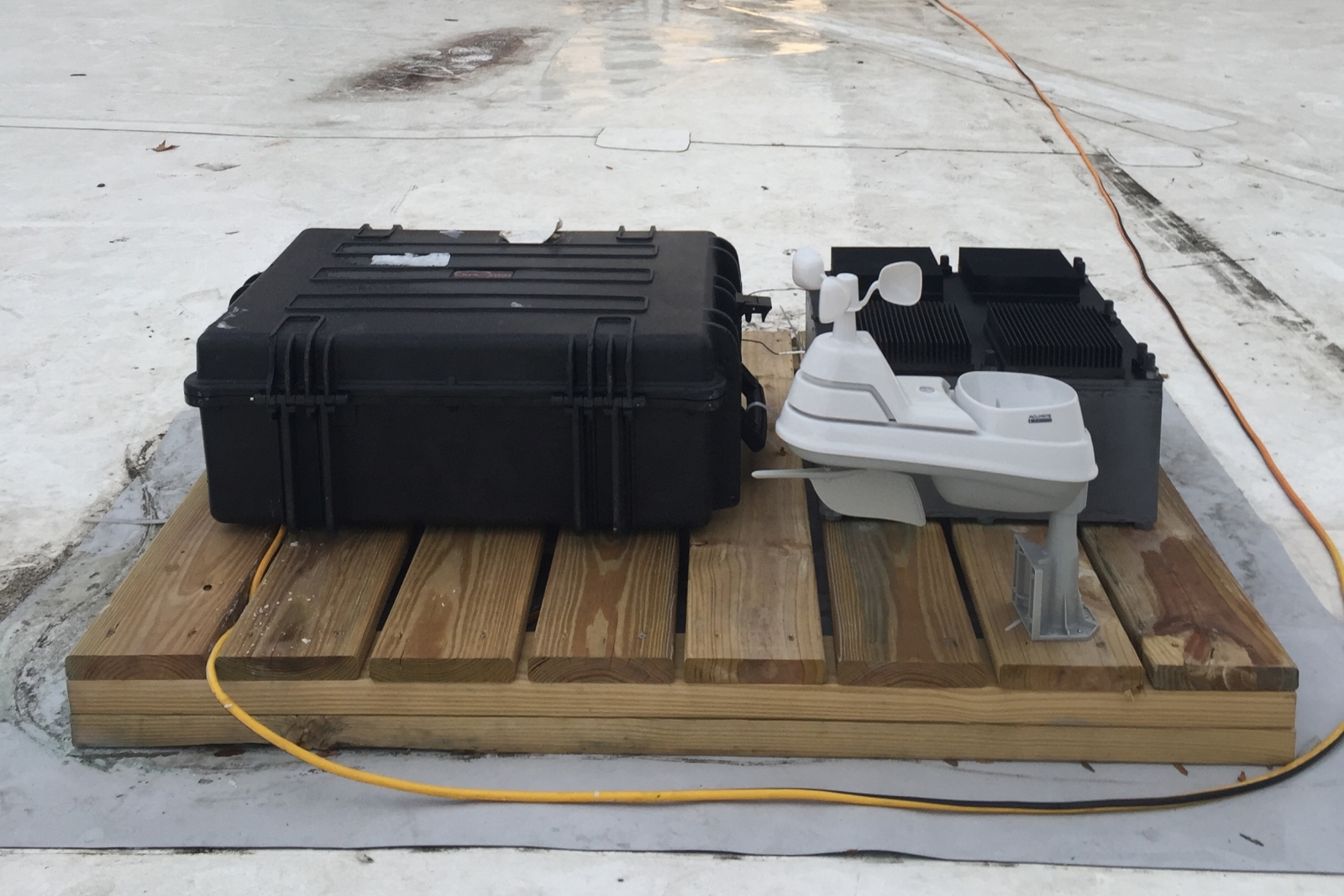
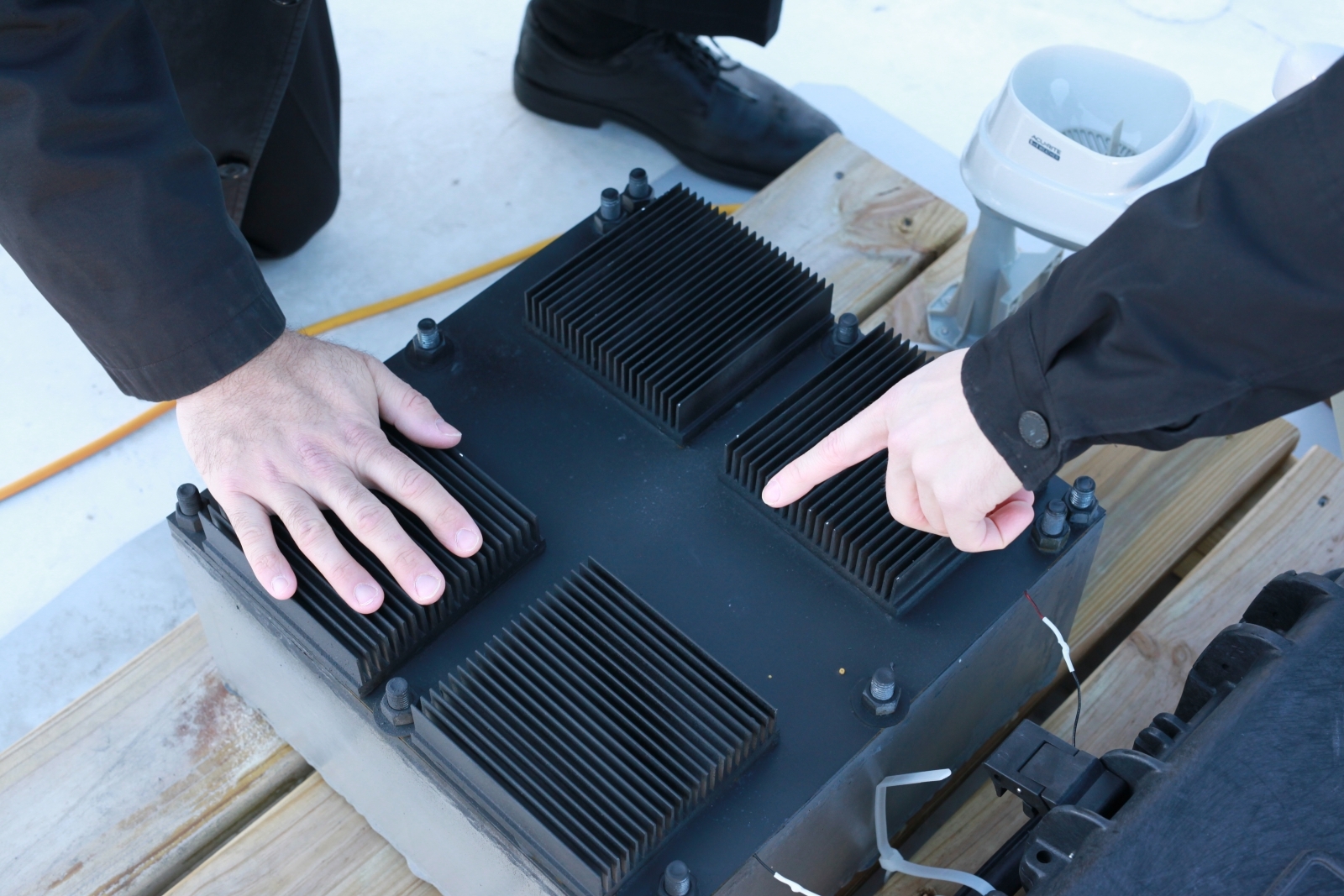
Engineers at the Massachusetts Institute of Technology (MIT) have created a device that can generate electricity out of what appears to be nothing but air. The device doesn't require sunlight, batteries or depend on wind, instead it relies on temperature fluctuations to generate energy.
The device, called a thermal resonator, was created using a combination of carefully customised materials.
"We basically invented this concept out of whole cloth," Michael Strano, a professor of chemical engineering at MIT, said in a statement. "We've built the first thermal resonator. It's something that can sit on a desk and generate energy out of what seems like nothing. We are surrounded by temperature fluctuations of all different frequencies all of the time. These are an untapped source of energy."
The material for such a system needs to be effective for a little-known characteristic called thermal effusivity – which describes how quickly the material can capture heat from its surroundings or release it. Thermal effusivity is the combination of thermal conduction and thermal capacity. While the former refers to how quickly heat can be distributed through the material, the latter refers to how much heat can be stored.
In most materials, such as ceramics, one of these properties is high, while the other is low – ceramics have low conduction but high thermal capacity. To circumvent this issue, the researchers created the system, whose basic structure is that of metal foam comprised of nickel or copper, coated with graphene. This allows for better thermal conductivity. The foam then infused the foam with a phase-changing, wax-like material called octadecane, which can transform into both solid and liquid within a specific temperature range.
"The phase-change material stores the heat and the graphene gives you very fast conduction," said MIT graduate student Anton Cottrill, the new study's lead author.
When a sample of the new material was tested, it was revealed that a mere 10 degree Celsius temperature change between night and day resulted in the material producing 350 millivolts of potential and 1.3 milliwatts of power. This is enough to power a small communications system or environmental sensors.
How does the device work? One side of the system gathers heat that slowly propagates through to the other side, while the other lags behind to reach equilibrium. According to Strano, the combination of the metal foam, the graphene and the octadecane makes it "the highest thermal effusivity material in the literature to date".
According to Volodymyr Koman, an MIT postdoc and the co-author of the new study, such systems could also provide long-lasting, low-power energy sources for space rovers exploring other planets.
Kourosh Kalantar-zadeh, a professor of engineering at RMIT University in Australia called such an approach a "novel development with a great future".
"It can potentially play an unexpected role in complementary energy harvesting units," he added. "To compete with other energy harvesting technologies, always higher voltages and powers are demanded. However, I personally feel that it is quite possible to gain a lot more out of this by investing more into the concept. It is an attractive technology which will be potentially followed by many others in the near future."
The new research, which has been published in the journal Nature Communications, was funded by a grant from Saudi Arabia's King Abdullah University of Science and Technology (KAUST). The Saudi university hopes to use the system to power networks of sensors that monitor conditions at oil and gas drilling fields.
The research also involved the work of seven other engineers including MIT chemical engineering graduate students Albert Tianxiang Liu, Amir Kaplan, and Sayalee Mahajan; visiting scientist Yuichiro Kunai; postdoc Pingwei Liu; and undergraduate Aubrey Toland and was supported by KAUST, the Office of Naval Research as well as the Swiss National Science Foundation.