Physicists use Einstein's 'spooky' entanglement to invent super-sensitive gravitational wave detector
Scientists have long been tried to come up with new and better detectors of gravitational waves.
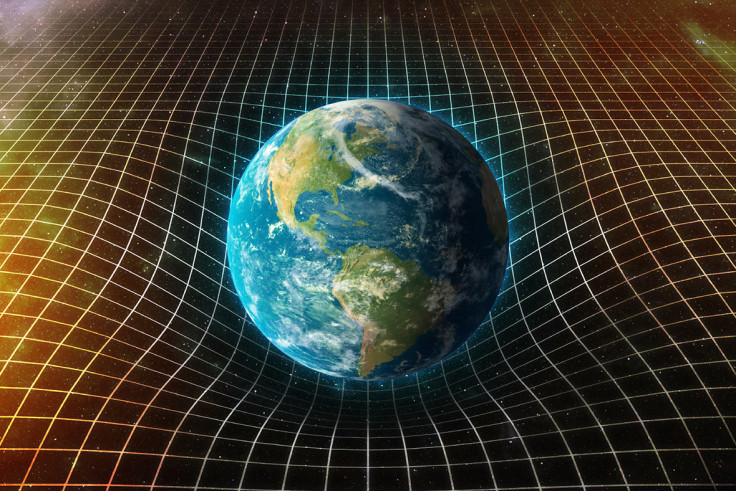
The first direct detection of gravitational waves, a phenomenon predicted by Einstein's 1915 general theory of relativity, was reported by scientists in 2016.
Armed with this "discovery of the century", physicists around the world have been planning new and better detectors of gravitational waves.
Last week physicists announced an exciting new design for gravitational wave detectors. The team was led by Yiqiu Ma of Caltech and Haixing Miao from Birmingham. It included their past PhD supervisor Chunnong Zhao from the University of Western Australia, and leading gravitational wave physicists Yanbei Chen and Belinda Pang from Caltech, Matthew Evans from MIT, Jan Harms from the University of Urbino, and Roman Schnabel from the University of Hamburg.
The new design is a real breakthrough because it can measure signals below a limit that was previously believed to be an insurmountable barrier. Physicists call this limit the standard quantum limit. It is set by the quantum uncertainty principle.
The new design, published in Nature magazine this week, shows that this may not be a barrier any longer.
Using this and other new approaches may allow scientists to monitor black hole collisions and "spacequakes" across the whole of the visible universe.
How gravitational wave detectors work
Gravitational waves are not vibrations travelling through space, but rather vibrations of space itself. They have already told us about an unexpectedly large population of black holes. We hope that further study of gravitational waves will help us to better understand our universe.
But the technologies of gravitational wave detectors are likely to have enormous significance beyond this aspect of science, because in themselves they are teaching us how to measure unbelievably tiny amounts of energy.
Gravitational wave detectors use laser light to pick up tiny vibrations of space created when black holes collide. The collisions create vast gravitational explosions. They are the biggest explosions known in the universe, converting mass directly into vibrations of pure space.
It takes huge amounts of energy to make space bend and ripple. Our detectors – exquisitely perfect devices that use big heavy mirrors with scarily powerful lasers – must measure space stretching by a mere billionth of a billionth of a metre over the four kilometre scale of our detectors. These measurements already represent the smallest amount of energy ever measured.
But for gravitational wave astronomers this is not good enough. They need even more sensitivity to be able to hear many more predicted gravitational "sounds", including the sound of the moment the universe was created in the big bang.
This is where the new design comes in.
A spooky idea from Einstein
The novel concept is founded on original work from Albert Einstein.
In 1935 Albert Einstein and co-workers Boris Podolsky and Nathan Rosen tried to depose the theory of quantum mechanics by showing that it predicted absurd correlations between widely spaced particles.
Einstein proved that if quantum theory was correct, then pairs of widely spaced objects could be entangled like two flies tangled up in a spider's web. Weirdly, the entanglement did not diminish, however far apart you allowed the objects to move.
Einstein called entanglement "spooky action at a distance". He was sure that his discovery would do away with the theory of quantum mechanics once and for all, but this was not to be.
Since the 1980s physicists have demonstrated time and again that quantum entanglement is real. However much he hated it, Einstein's prediction was right and to his chagrin, quantum theory was correct. Things at a distance could be entangled.
Today physicists have got used to the "spookiness", and the theory of entanglement has been harnessed for the sending of secret codes that cannot be intercepted.
Around the world, organisations such as Google and IBM and academic laboratories are trying to create quantum computers that depend on entanglement.
And now Zhao and colleagues want to use the concept of entanglement to create the new gravitational wave detector's design.
A new way to measure gravitational waves
The exciting aspect of the new detector design is that it is actually just a new way of operating existing detectors. It simply uses the detector twice.
One time, photons in the detector are altered by the gravitational wave so as to pick up the waves. The second time, the detector is used to change the quantum entanglement in such a way that the noise due to quantum uncertainty is not detected.
The only thing that is detected is the motion of the distant mirrors caused by the gravitational wave. The quantum noise from the uncertainty principle does not appear in the measurement.
To make it work, you have to start with entangled photons that are created by a device called a quantum squeezer. This technology was pioneered for gravitational wave astronomy at Australian National University, and is now an established technique.
Like many of the best ideas, the new idea is a very simple one, but one that took enormous insight to recognise. You inject a miniscule amount of squeezed light from a quantum squeezer, and use it twice!
Around the world physicists are getting ready to test the new theory and find the best way of implementing it in their detectors. One of these is the GEO gravitational wave detector at Hannover in Germany, which has been a test bed for many of the new technologies that allowed last year's momentous discovery of gravitational waves.
This article was modified on 21 May to better describe the international team that made this discovery.
David Blair, Director, WA Node of the ARC Centre of Excellence for Gravitational Wave Discovery, and the Australian International Gravitational Research Centre, University of Western Australia
This article was originally published on The Conversation. Read the original article.
© Copyright IBTimes 2025. All rights reserved.